By Patrik Kalbermatten, Senior Manager – Distribution Promotion Product Management Magnetic, Sensor & Actuator at KEMET Electronics Corporation a YAGEO company
Piezoelectric actuators that exploit the inverse piezoelectric effect to produce a displacement in response to an applied voltage can offer an alternative to familiar electromagnetic devices such as motors and solenoids. They offer advantages such as greater reliability, lower power consumption, smaller size, and increased positional resolution.
The piezoelectric effect
The Curie brothers demonstrated the direct piezoelectric effect at the end of the 19th century, showing how applying stress to naturally occurring crystalline materials such as quartz produces an electric charge (figure 1a). There is also an inverse effect: applying an electric field to a material with piezoelectric properties causes a physical distortion (figure 1b), resulting in a displacement of a few microns.
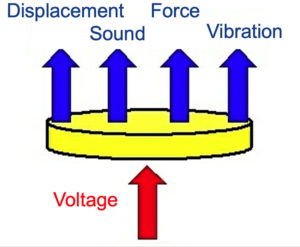
Since the Curies’ research, various synthetic piezoelectric materials, or ferroelectric ceramics, have been developed. These typically have a much higher piezoelectric constant than natural materials. After sintering, the dipoles within the crystalline structure are randomly oriented. A strong electric field is then applied to polarize the dipoles. A residual polarization persists after this field is removed, giving the ceramic its piezoelectric properties.
Among these synthetic materials, PZT, or lead zirconate titanate (Pb (Zr, Ti) O3), has high sensitivity and a high operating temperature that enable use in practical applications. Its direct piezoelectric properties are exploited in devices such as sensors for pressure, vibration, acceleration, and shock, ultrasonic receivers and diagnostic equipment, sonar instruments, fish finders, non-destructive testing equipment, and microphones.
On the other hand, leveraging the inverse piezoelectric effect to manipulate the crystal dimensions enables actuators such as precision industrial positioning stages, valves, camera-lens motors for zoom and auto-focus, ultrasonic sources, and loudspeakers to be created.
Piezoelectric actuator types and construction
Various types of actuators can be constructed to produce different types of movement by applying a voltage in a longitudinal or transverse direction to cause displacement. Figure 2 compares longitudinal, transverse, and stacked longitudinal elements, showing the direction of displacement, and a bimorph transverse element that produces a bending displacement.
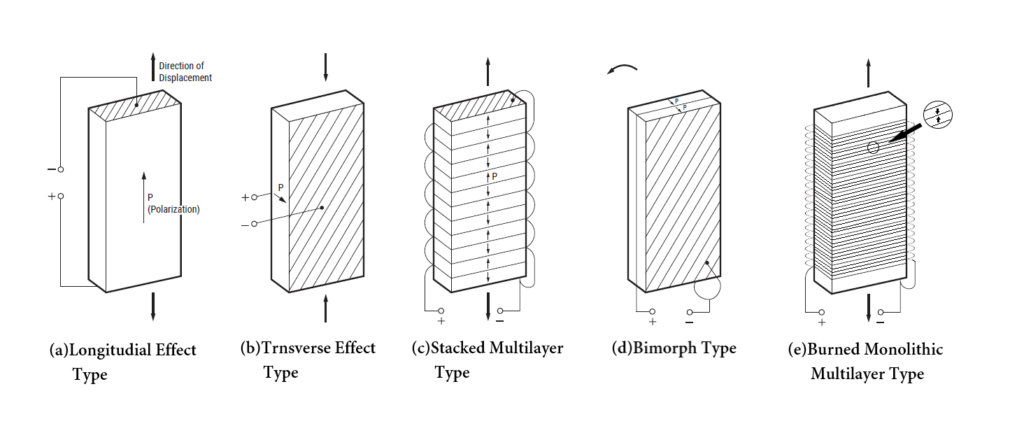
The longitudinal effect type requires a large voltage to obtain displacement because the electrode spacing is long. The transverse effect type can reduce the voltage because the electrode spacing can be shorter than the longitudinal effect type, but the displacement amount is smaller because the displacement in the direction perpendicular to the polarization direction is used. The stacked type has a short electrode spacing and uses displacement in the polarization direction, so displacement can be obtained with a low voltage, but there is the inconvenience of having to stack each piezoelectric ceramic. In addition, the bimorph type can obtain a large displacement at a low voltage, but since it uses the displacement in the bending direction, a large generated force cannot be obtained, and there is a problem in the durability of repeated driving.
Integrally fired multilayer piezoelectric actuators overcome these issues by making the space between the electrodes extremely thin, thus achieving a large displacement at a voltage that is sufficiently low for practical use.
Features of the piezoelectric actuator
Compared to electromagnetic actuators, such as small electric motors or solenoids that could be used to create precisely controlled movements, piezoelectric actuators have a number of advantages. The response time is extremely fast. In addition, the actuator produces no electromagnetic noise, which can simplify the system design, eliminate shielding and filtering components from the bill-of-materials, and ease EMC compliance testing. Thermal management can also be greatly simplified since minimal heat is generated. Compactness and lightweight are further advantages and piezoelectric actuators allow precise control with high resolution. The table compares the key aspects that should be considered when designing with electromagnetic and piezoelectric actuators.
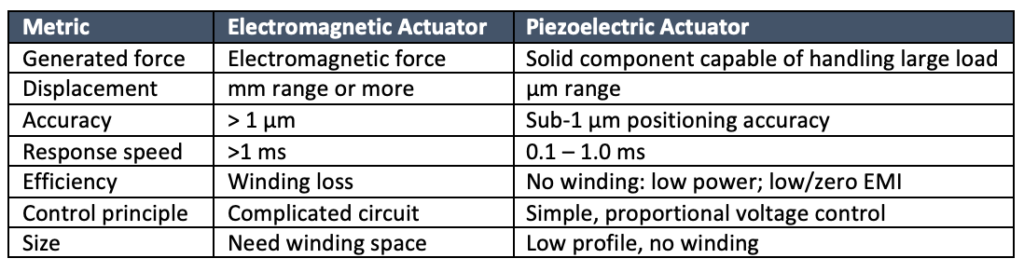
Multilayer piezoelectric actuators
Despite having generally excellent characteristics, some care is recommended when designing with multilayer actuators. Repetitive actuation can cause the element to break as the non-active insulating area between positive and negative internal electrodes expands and contracts differently compared to the active areas, introducing mechanical stresses. “Full electrode” actuators are better able to withstand repetitive actuation. Extending the electrode across the full width of each plate, and introducing a glass insulator between the electrodes (Figure 3), eliminates the non-active area and so prevents the stresses caused by differential expansion.
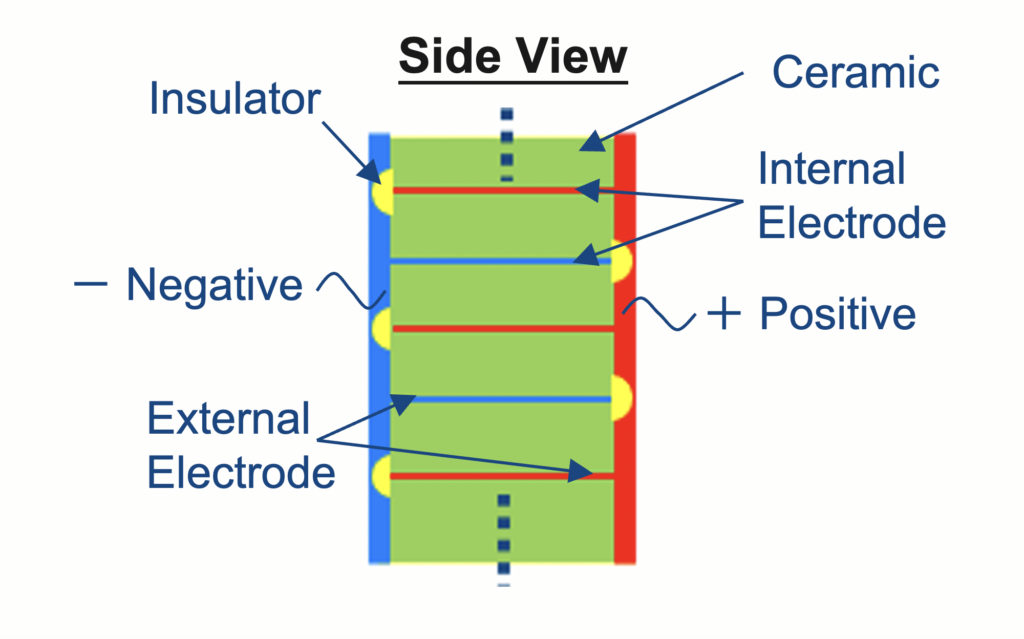
Also, environmental challenges such as high humidity can shorten the actuator’s lifetime. Hermetically sealed actuators can offer enhanced reliability in applications that are exposed to harsh environments. These may contain a high-displacement piezo material housed in a completely sealed metal case (figure 4). The case has a pre-loaded bellows structure that can expand and contract with the element and may provide a metal flange to simplify mounting.
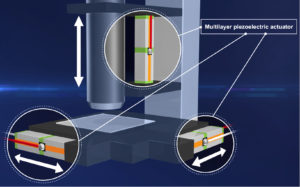
Applications
The change in dimension of the piezoelectric element can be used to produce a linear displacement for various effects such as operating pumps, valves, and controlling precision positioning mechanisms. Figure 5 shows how piezoelectric actuators installed on three axes can control the mechanisms for x-y stage position and lens focus in a precision inspection instrument.
Figure 6 shows how a piezoelectric actuator can control the dispensing of adhesive and ensure precision metering suitable for high-speed assembly of products such as smartphones.
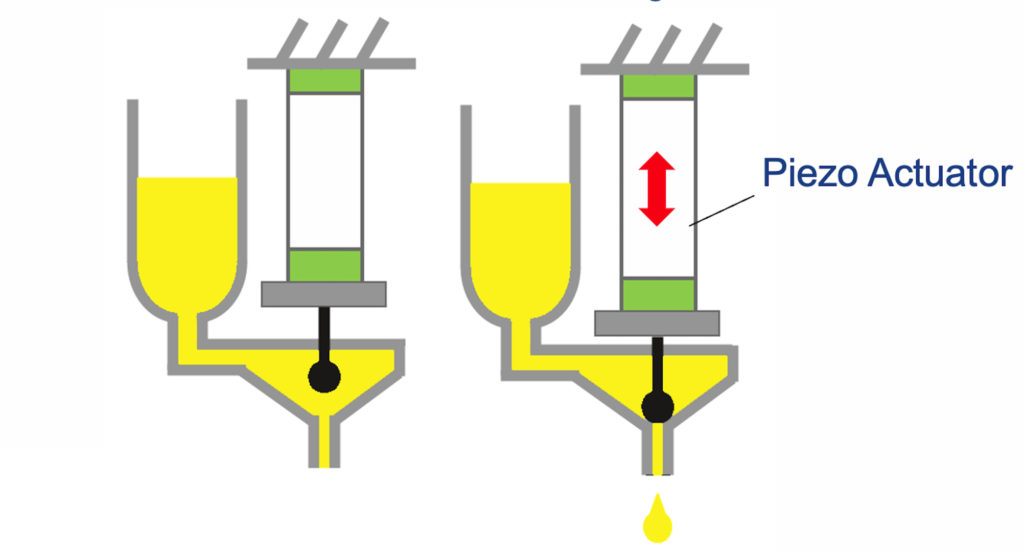
Resin coated ring-shaped actuators are suitable for positioning applications such as fine-tuning laser wavelength and phase in high-precision Fabry-Perot resonators for applications such as interferometry (figure 7). Controlling the expansion and contraction of the piezoelectric actuator allows the wavelength of the laser to be varied.
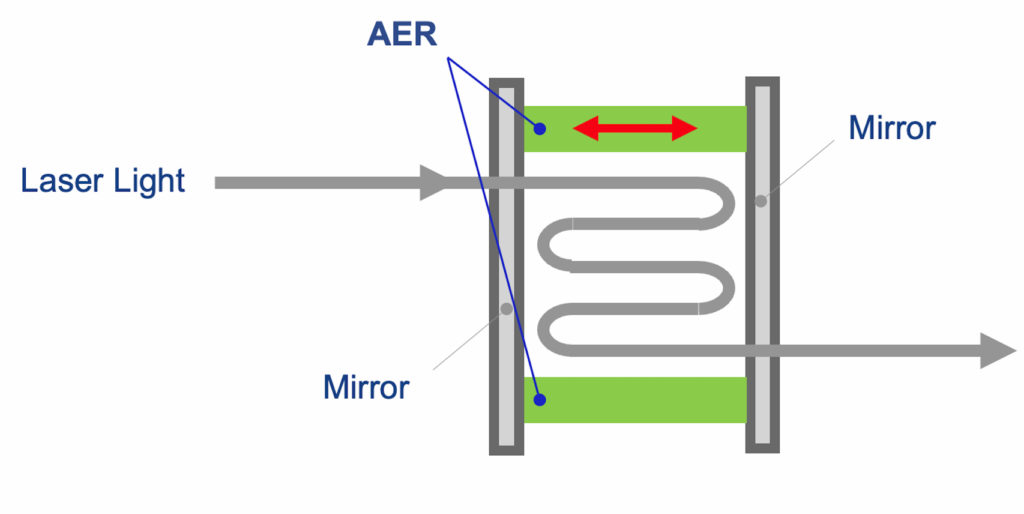
Figure 8 shows how the change in length of the actuator can be utilized in a mass flow controller. For film formation equipment and etching equipment used in semiconductor manufacturing, these actuators provide ultra-precise gas flow control in such mass flow controllers.
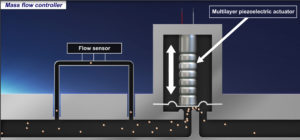
Enhancing performance and reliability
With minimal moving parts, and leveraging features such as multilayer, full-electrode construction, piezoelectric actuators deliver inherent reliability benefits over alternatives such as electromechanical actuators. To maximize their reliability and performance, users can consider some simple design guidelines. When designing the fixing, prevent the actuators from being bent, twisted, or subjected to tensile force. As a rule of thumb, any twisting force should be less than 3 × 10-1 N・m for an actuator that generates a force of (compression resistance) of 800 N. Tensile force should be limited to 50 N or less. The actuator should be installed so that the centre axis of the generated displacement is aligned with the centre axis of the load.
Regarding driving the actuator, the amount of displacement is approximately proportional to the applied voltage. A controller is needed to generate the required voltage pattern and drives the actuator through an amplifier that produces the necessary drive voltages. In high-precision positioning applications, closed-loop feedback can enhance control over displacement. Reverse voltages should not be applied.
Hysteresis, ringing, creep, and other similar phenomena should be considered when designing the driving circuit. To prevent intensive ringing, which can cause the actuator to break, the rise or fall of the applied voltage should be limited to below 1/3 of the resonance frequency of the actuator element.
The actuation of the piezoelectric actuator is similar to the injection of electric charges into a relatively large capacitor. A large electric current is necessary to realize the high-speed response of the actuator. For pulse driving, the driver design should consider self-heat generation, charge/discharge current, and the power supply’s impedance.
Conclusion
Piezoelectric actuators permit simple and precise motion control, bringing benefits such as low power consumption, low noise, and compact dimensions to industrial, medical, and consumer products. Multilayer, full-electrode stacked actuators produce a large displacement in relation to the applied voltage and are highly resistant to the stresses of repeated actuation.