It generates quantum entanglement between a microwave resonator and a signal that is emitted toward a target and can theoretically make radar detection up to four times faster in scenarios with comparable signal power and target noise. It also reduces susceptibility to jamming.
A microwave quantum radar begins with a stream of entangled visible-frequency photons created using a superconducting Josephson parametric converter (JPC). The entangled photons are sent through a beam splitter to create two beams, the signal beam and the idler beam. The signal beam is converted to microwave frequencies and the entangled quantum state is maintained. The signal beam is then used as a conventional radar beam, sent, and received to detect a target. The returned signal beam is converted back into visible photons, and the detector compares the returned signal photons with the photons in the idler beam (Figure 1).
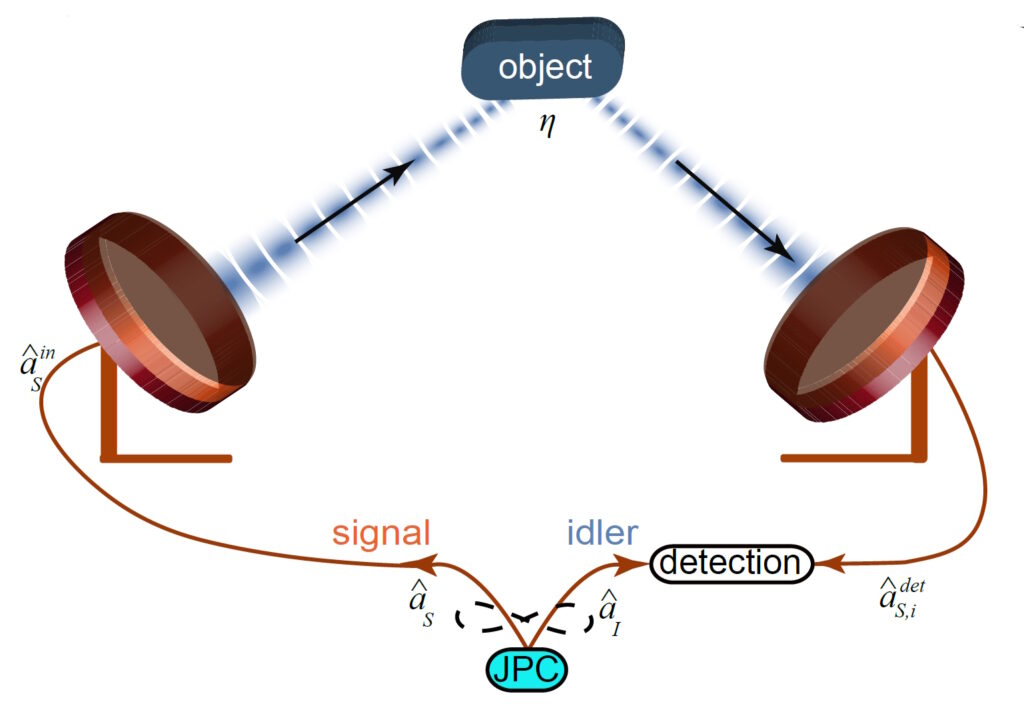
Quantum entanglement is fragile, and a lot is lost when the signal beam is reflected. However, the correlation between the signal and idler photons remains strong enough to separate them from background noise. Conventional radar is highly susceptible to background noise and overcomes that problem by using high-power transmissions. Theoretically, a quantum radar can provide superior performance with much lower power transmissions, making them difficult or impossible for an adversary to detect.
In addition, conventional radar can be made ineffective if jamming signals are transmitted on the same frequency that the radar is using. Without any way to distinguish the radar signal from the jamming signal, the system can’t identify targets. In the case of a quantum radar, the adversary can’t match the entanglement of the signal and idler beams and the system can operate robustly even in the presence of jamming signals.
Biomedical scanning is another potential application for quantum radar. Since it can operate effectively with low levels of radiation, it may be useful as a non-invasive scanning method for human tissues or proteins.
While quantum radar can theoretically outperform conventional radar by a factor of four or more, fabricating an operating system has proven challenging. Progress has recently been realized in the pursuit of a working quantum radar system.
A superconducting quantum radar circuit that operates at cryogenic temperatures has been fabricated and achieved a 20% performance improvement compared to conventional radar. The circuit creates the quantum entanglement between the signal and idler beams and includes the detection circuitry for target identification using an integrated microwave photon counter for the returned entangled microwave signal (Figure 2).
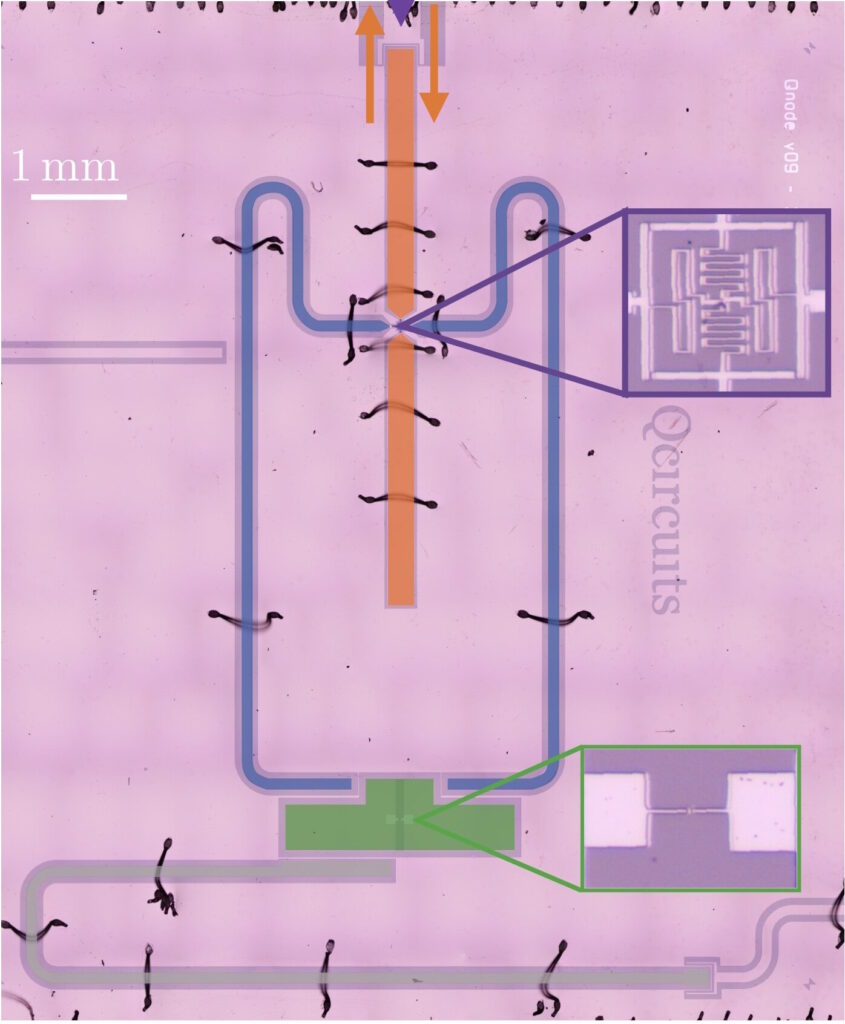
Summary
Quantum radar uses entangled photons to improve target detection in noisy environments. It can operate effectively at lower power levels than conventional radar. Its low power operation makes it difficult for an adversary to detect and potentially makes the technology suitable for applications in biomedical systems.
References
A quantum radar that outperforms classical radar by 20%, Phys.org
A Study on Quantum Radar Technology Developments and Design Consideration for its integration, arVix
Advantages and Limitations of Quantum Radar, IEEE
How Quantum Radar Could Completely Change Warfare, Popular Mechanics
Microwave quantum illumination using a digital receiver, arVix
Quantum radar, Wikipedia
Quantum radar has been demonstrated for the first time, MIT Technology Review